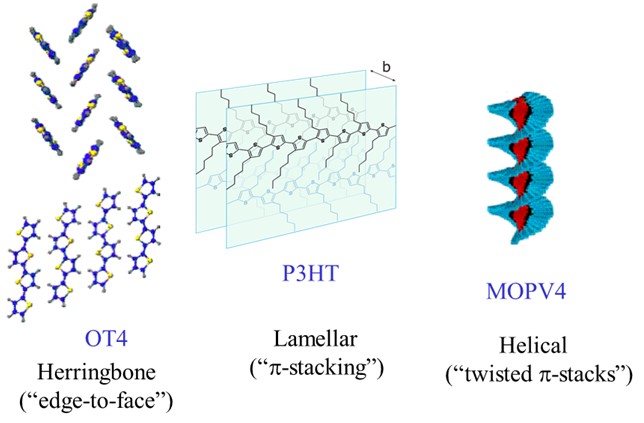
Figure 1. Variety of packing architectures for conjugated molecules and polymers.
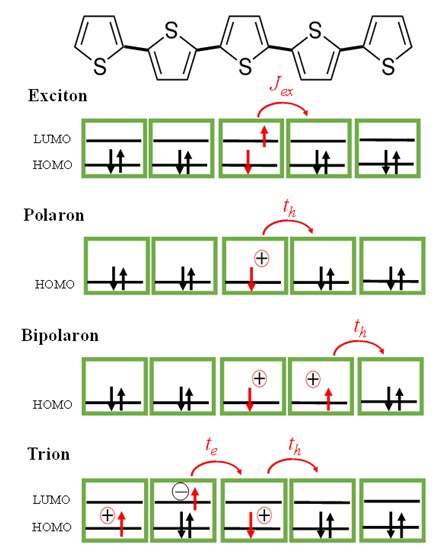
Figure 2. Simplified view of various excitations.
Research Description
The Spano Group is primarily interested in modelling the photophysical properties of organic materials, such as molecular aggregates, thin films and crystals comprised of small p-conjugated organic molecules such as tetracene as well as large semiconducting polymers, like polythiophene. Such materials can assemble in a remarkable number of ways – Figure 1 shows but a few. They can be fashioned into solar cells, light emitting diodes and sensors for a range of commercial applications. The interactions which drive charge and energy transport necessary for device operation can be revealed through the shape of the absorption and photoluminescence spectra, i.e. through spectral “signatures”. The group is particularly interested in how the complex interplay of long-range Coulomb exciton couplings, charge transfer, vibronic coupling, and disorder can be unraveled through the interpretation of well-defined spectral signatures.
In organic materials the simultaneous presence of electronic, vibrational and even photonic degrees of freedom (for example, in optical microcavities) are responsible for the creation of a variety of quasiparticles such as excitons, excimers, polarons, bipolarons, trions and polaritons which contribute in various ways to the optoelectronic response. Figure 2 presents a simplified depiction of some quasiparticle excitations in a polythiophene chain, where each chromophoric unit is a single thiophene unit. In neutral P3HT excitons coupled to intrachain vibrations are responsible for a pronounced vibronic progression in the visible region of the absorption spectrum as well as in the photoluminescence spectrum, details of which are very sensitive to the intermolecular interactions present in aggregates. In oxidized chains, polarons, bipolarons and trions lead to distinctive features in the mid- and near-IR regions of the absorption spectrum, which yield important information about the particle’s wave function.
Fundamental Theory of Excitons and Excimers
Excitons, which are comprised of a bound electron/hole pair, are ubiquitous in organic and inorganic semiconductors. In organic molecular aggregates and crystals, the excitons are very tightly bound such that the electron and hole reside on the same chromophore; in this case the electron is viewed as a half-filled LUMO and the hole as a half-filled HOMO. Such excitons are called Frenkel excitons and can effectively transport energy, as required, for example, in a solar cell.
The optical response of Frenkel excitons have fascinated scientists since their discovery in the 1930s. A great deal of research has been directed at determining how the properties of such excitons – such as the binding energy, exciton bandwidth, coherence length, etc. – can be determined through absorption and photoluminescence spectroscopy. The classification of H-aggregates and J-aggregates by Michael Kasha in the 1950s was a significant step forward. Here, the sign of the exciton coupling – which can then related to the way molecules are packed in the solid phase – is determined from spectral shifts. In J-aggregates (H-aggregates) the main absorption peak is red-shifted (blue-shifted) relative to an unaggregated monomer.
Our unique approach to understanding Frenkel excitons involves vibronic signatures – those which derive from the coupling between the excitons and vibrations. For a great many conjugate organic molecules the quinoidal-aromatic vibrational mode with an energy of approximately 1400 cm-1 is the most strongly coupled, leading to pronounced vibronic progressions in isolated (unaggregated) molecules in solution. However, when molecules interact electronically, the vibronic progression is distorted in a manner which depends on the sign of the electronic coupling. In H-aggregates, the first vibronic peak in the absorption spectrum is attenuated relative to the second peak, by an amount which depends directly on the strength of the coupling. The opposite holds for J-aggregates; here the first peak is enhanced relative to the second peak. Figure 3 shows how the absorption and emission spectra of H- and J-aggregates respond to increasing exciton bandwidth, W.
We have also expanded the Frenkel-Holstein approach to encompass intermolecular charge transfer (CT), where electrons and holes can liberate themselves from the confines of Frenkel excitons – see Figure 4. Frenkel exciton dissociation into a CT state in which the electron and hole reside on neighboring chromophores, is an important process in molecular p-stacks, for example. The Frenkel-CT Holstein Hamiltonian has been quite successful in describing the photophysics of perylene diimide p-stacks as well as polyacene (tetracene, pentacene) molecular crystals.
Current and future research concerns the development of models which include not only the intramolecular vibrations but also intermolecular vibrations which are involved in nonlocal exciton-vibrational coupling. The latter leads to the formation of excimers, in which the excited state is subject to relaxation along an intermolecular coordinate, such as an intermolecular twist, resulting in a generally red-shifted emission spectrum with a significant loss of vibronic structure. One main goal is to accurately identify which types of intermolecular packing leads to efficient excimer formation.
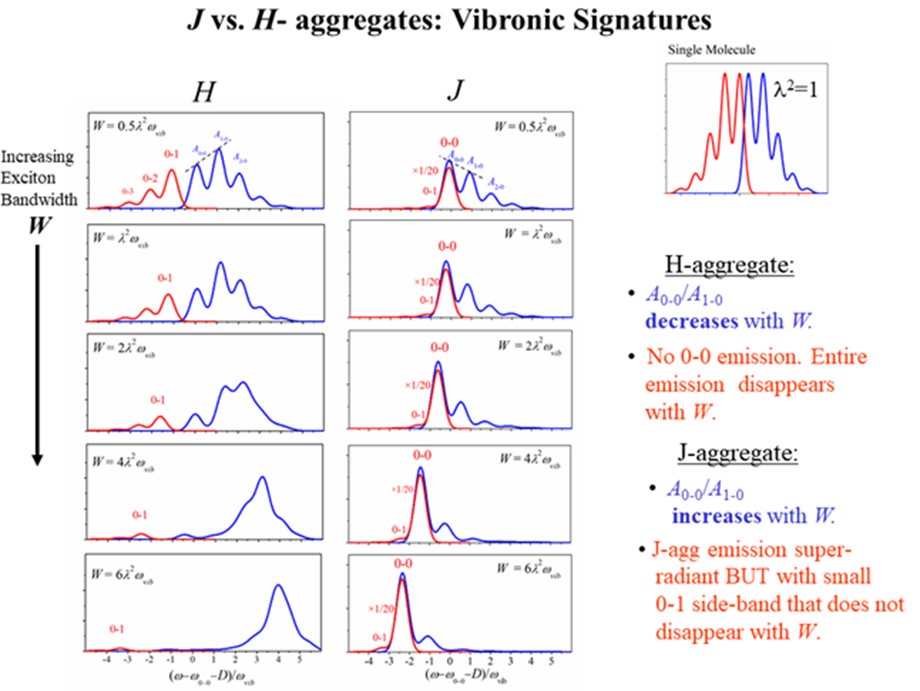
Figure 3. Spectral signatures of H- and J-aggregates. Adapted from F.C. Spano, Acc. Chem Res, 43, 429-439 (2010).
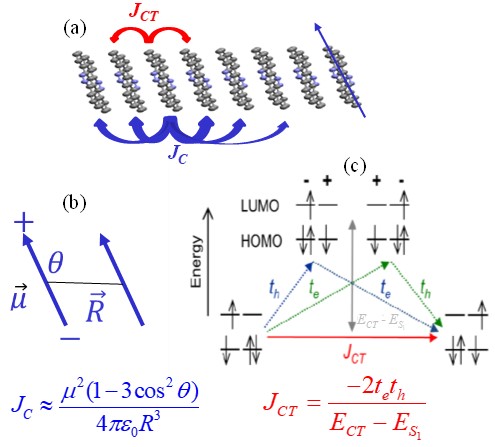
Figure 4. a) pi-stack depicting long-range Coulomb interactions, JC, and short-range CT-mediated interactions, JCT. The Coulomb interactions derive from interacting transition dipoles in b) while CT-mediated interactions derive from a transfer of an electron and hole through a virtual intermediate CT state. te and th are the electron and hole transfer integrals respectively. Adapted from Hestand and Spano, Acc. Chem. Res. 50, 341-350 (2017).
Some Relevant Papers:
- J. Hestand and F.C. Spano, Expanded Theory of Molecular J- and H-aggregates: The Effects of Vibronic Coupling and Intermolecular Charge Transfer, Chem. Rev. 118, 7069-7163 (2018).
- C. Spano, The Spectral Signatures of Frenkel Polarons in H- and J-aggregates, Acc. Chem. Res. 43, 429-439 (2010).
- J. Hestand and F. C. Spano, Molecular Aggregate Photophysics Beyond the Kasha Model: Novel Design Principles for Organic Materials, Acc. Chem. Res., 50, 341-350 (2017).
- A. Oleson, T. Zhu, I. S. Dunn, D. Bialas, Y. Bai, W. Zhang, M. Dai, D. R. Reichman, R.Tempelaar, L. Huang, F. C. Spano, Perylene Diimide-Based Hj- and hJ-Aggregates: TheProspect of Exciton Band Shape Engineering in Organic Materials, J. Phys. Chem. C, 123, 20567-20578 (2019).
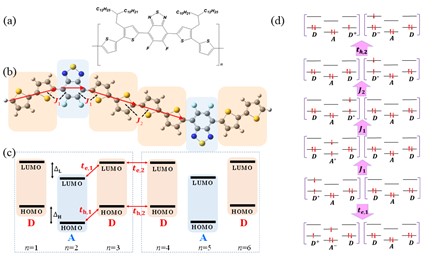
Figure 5. Low bandgap donor-acceptor polymers. Adapted from, M.B. Qarai et. al. J. Chem. Phys. 153, 244901, (2020).
Some Relevant Papers
M. B. Qarai, Xin Chang, and F. C. Spano, Vibronic exciton model for low band-gap donor-acceptor polymers, J. Chem. Phys., 153, 244901 (2020).
Yamagata, F.C. Spano, “Strong Photophysical Similarities between Conjugated Polymers and J-aggregates”, J. Phys. Chem. Lett. 5, 622-632, 2014.
Vibronic Frenkel-CT Exciton Model for Conjugated Polymers
We have also applied the Frenkel-CT Holstein approach to individual polymer chains where a repeat unit can be viewed as the unit chromophore coupled to its immediate neighbors mainly via electron and/or hole transfer. We are interested in the spectral response of excitons in homopolymers like P3HT which we found behaves in many ways like a linear J-aggregate due to an in-phase relationship between electron and hole transfer integrals (based on translational symmetry) as well as aligned (heat-to-tail) transition dipole moments. Interestingly, because interchain interactions are “H-like” we discovered that p-stacks of P3HT behave like “HJ”-aggregates where the intrachain J-promoting couplings compete with interchain H-promoting couplings in determining the optical response.
We are currently pursing H, J and HJ aggregates in low bandgap donor acceptor polymers – see Figure 5- which show the greatest promise for commercial applications.
Polarons, Bipolarons and Trions in Conjugated Polymers
In addition to neutral excitations there are a host of charged excitations in conjugated molecule/polymer chains and aggregates which arise via oxidation or reduction. The most celebrated examples include chemically doped polymers like P3HT in which strong electron withdrawing molecules like F4TCNQ introduce “holes” into the polymer chain. Such holes are strongly coupled to the nuclear framework and are more accurately described as positive polarons. Bound polaron pairs or bipolarons are suspected of having an important role in conductivity at higher dopant concentrations. Our research uses a Holstein-style Hamiltonian to probe the structure of polarons and bipolarons in conjugated polymers. Thus far the mid-IR spectrum for single polarons in P3HT has been accounted for with considerable success, and we are expanding our investigation to include bipolarons and trions which are active in the mid to near-IR spectral region.
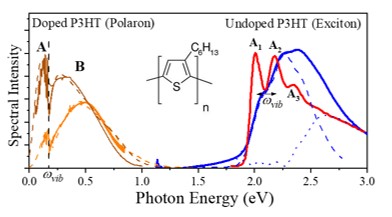
Figure 6. Mid-IR and UV-vis of doped and undoped P3HT respectively. (Dotted curves are our simulations). Adapted from Ghosh and Spano, Acc. Chem. Res. 53, 2201, (2020).
Some Relevant Papers
- Ghosh and F. C. Spano, Excitons and polarons in organic materials, Acc. Chem. Res. 53, 2201-2211 (2020).
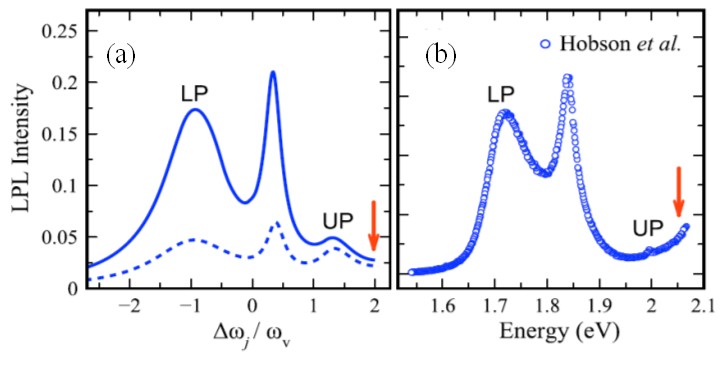
Figure 7. a) The leakage PL calculated for a microcavity containing 20 chromophores b) The experimental PL spectrum for a J-aggregate immersed in a tuned microcavity. (Hobson, et. al. Appl. Phys. Lett. 81, 3519-3521 (2002). Figure adapted from Herrera and Spano, PRL, 118, 223601, (2017).
Some Relevant Papers
- Herrera and F.C. Spano, “Cavity-Controlled Chemistry in Molecular Ensembles”,Phys. Rev. Lett., 116, 238301, 2016.
Herrera and F. C. Spano, “Dark Vibronic Polaritons and the Spectroscopy of Organic Microcavities”, Phys. Rev. Lett., 118, 223601 (2017).
Polaritons in Organic Microcavities
Inserting organic chromophores in between two optical mirrors spaced approximately a visible wave length apart leads to the creation of exotic particles called polaritons which are composed of a material excitonic part as well as a radiation (or photonic) part. Many experiments are being performed to see if polariton formation can affect the outcome of chemical reactions with some impressive results so far. We are interested primarily in the types of qausi-particles that are formed from the combination of electronic, vibrational and photonic degrees of freedom. In collaboration with Prof Felipe Herrera at the University of Santiago, we have discovered a new type of “dark” polariton which is an eigenstate of the so-called Holstein Tavis Cummings Hamiltonian. Dark polaritons are quite unusual because they possess no oscillator strength to the vibrationless ground state but nevertheless couple strongly to the cavity field. As shown in Figure 7 they are essential for understanding the photoluminecence spectrum. We further showed how photo-induced electron-transfer reaction can be controlled using an optical microcavity appropriately tuned to the optical transition of the donor. The cavity-donor coupling is able to reduce the Marcus reorganization energy via vibronic decoupling, thereby enhancing the reaction rate.